Article 3: Grasping Carbon Dioxide Flow Physics. Part 1
Understanding the dynamics of fluid flow, whether in the context of hydrocarbon reservoirs or CO2 storage, is paramount for predicting subsurface flow behaviour accurately. While CO2 can be injected as part of enhanced oil recovery into depleted oil and gas fields, geological CO2 storage entails injection into saline aquifers and depleted hydrocarbon reservoirs. In the realm of multiphase flow theory, the introduction of non-wetting fluid (CO2) into a water-wet porous medium (brine) constitutes a drainage process, contrasting with the imbibition (water uptake) process observed in oil field waterflooding.
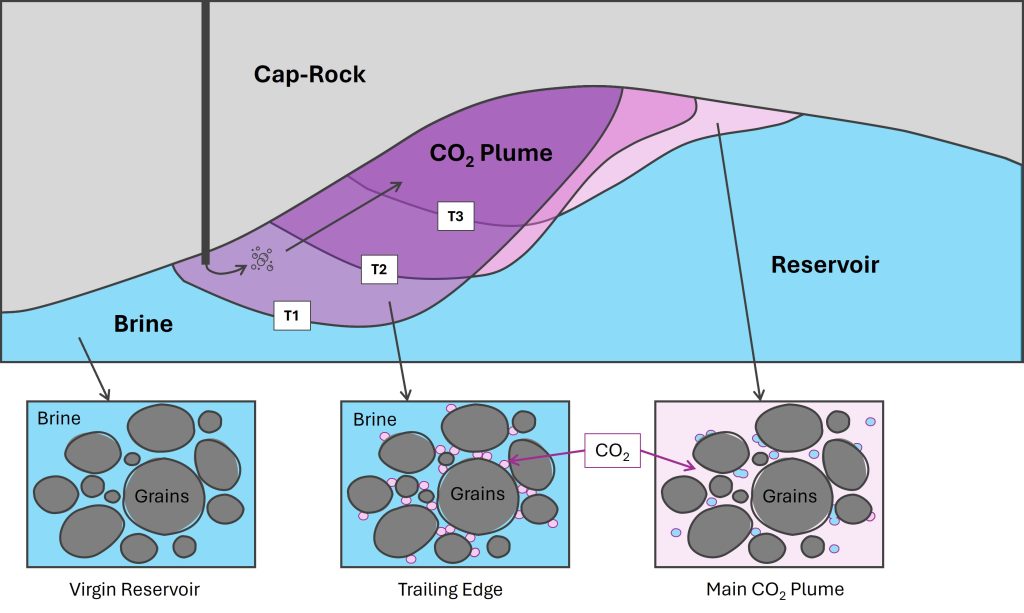
As CO2 is injected and migrates through the formation, both drainage (plume front) and imbibition cycles (brine filling behind the rising plume) occur, with drainage being the first to happen. This process bears resemblance to geological filling processes of oil in a reservoir over extended timeframes, unlike the rapid hydrocarbon production typical of oil and gas extraction over what we call a production timescale. Also, due to its similar density to oil, liquid CO2 rapidly exhibits gravity-dominated flow characteristics as it drains into the water-wet reservoir, with viscous forces diminishing away from the wellbore region.
In fluid dynamics, capillary, gravity, and viscous forces are primary influencers of flow dynamics, with one force often dominant over the others. Traditional reservoir simulators, designed for viscous-dominated flows (Darcy), are now being adapted for CO2 modelling in Carbon Capture, Utilisation, and Storage (CCUS) projects. However, extensive research indicates that CO2 flow is primarily governed by capillary forces across much of the storage space. Capillary pressure refers to the pressure difference between two immiscible fluids (like CO2 and brine) in a small pore space, influenced by surface tension and pore geometry.

The pressure difference between CO2 and brine has significant implications for reservoir flow simulation in CO2 storage projects, necessitating higher-resolution grids and more frequent time-stepping to capture multiphase flow dynamics accurately. Moreover, model sizes for saline aquifers can notably surpass those needed for oil and gas projects, particularly when accounting for pressure influences on or from regional aquifers. Additionally, the temperature dependence of CO2 properties mandates non-isothermal simulations. This is crucial in shallower reservoirs, where conditions approach the CO2 critical point, to avoid underpredicting the buoyancy forces.
Furthermore, the need to evaluate CO2 dissolution in the brine phase, and various geochemical processes, including salt drop-out, may necessitate the use of reaction-geochemistry models. Fortunately, simulation tools have evolved to address these challenges. Coupled with high-performance and massively parallelised computing frameworks, they enable efficient forecasting of complex CO2 storage scenarios.
The interplay between buoyancy and capillary forces dictates CO2 movement post-injection, with viscous forces typically negligible due to low flow rates. This is because pressure gradients fall to hydrostatic levels within 10s to 100s of meters of the wellbore, resulting in very low flow rates and negligible viscous forces (Bebb et al., 2021). In fact, in most cases, the rate of CO2 injection at wells is unlikely to exceed the boundary conditions for capillary-dominated flow (Bebb et al., 2021). This means that most of the storage domain during and after injection is dominated by buoyancy and capillary forces, and not by viscous-dominated forces (Bebb et al., 2021).
For geologists who are occupied with understanding geological reservoir architecture and sedimentary basin history, their skills may be even more relevant to CO2 storage projects than they were for hydrocarbon reservoir studies. This is because geological heterogeneity has a strong effect on the capillary- and gravity-dominated flow processes characteristic of CO2 storage projects (Reynolds & Krevor 2015; Trevisan et al. 2017). For example, the Sleipner CO2 plume has pronounced layering due to vertical heterogeneity of the storage unit (Furre et al. 2017). In contrast, the viscous-dominated flow regimes of gas and oil production systems are less affected by rock heterogeneity (Bentley & Ringrose, 2021).
Conclusions
Flow dynamics must be understood in the context of capillary, gravity and viscous forces in order to accurately predict subsurface flow of an injected CO2 plume. Current research suggests that the dominant force is capillarity i.e. the pressure difference between CO2 and brine in a restricted pore space and under the influence of surface tension and pore geometry. This multi-phase behaviour must be adequately captured by reservoir flow simulators, necessitating higher-resolution grids and more frequent time-stepping. Geological heterogeneity can also strongly influence flow of the CO2 plume, highlighting the need for close integration between reservoir engineers and geologists when modelling CO2 flow in the subsurface.
Next week’s article will dive deeper into the flow physics of CO2, specifically concentrating on invasion percolation.
Benjamin Adillah, Senior Reservoir Engineer
References
- Bebb, F, 2021, Understanding Flow Dynamics of CO2 Plumes in the Subsurface. Subsurface Insights, February 2021, p. 21–25.
- Bebb, Florence Letitia, Evans, Kate Clare Serena, Mukherjee, Jagannath, Saeed, Bilal, and Geovani Christopher. “Understanding the Flow Dynamics of CO2 Plumes in the Subsurface.” Paper presented at the Abu Dhabi International Petroleum Exhibition & Conference, Abu Dhabi, UAE, November 2021. Doi: https://doi.org/10.2118/207539-MS.
- Bentley, M. and Ringrose, P. [2021]. The Rock Model. In Reservoir Model Design (pp. 11-63). Springer, Cham.
- Carruthers, D., and P. Ringrose, 1998, Secondary oil migration: Oil-rock contact volumes, flow behaviour and rates, in J. Parnell, ed., Dating and duration of fluid flow and fluid-rock interaction: Geological Society (London) Special Publication 144, p. 205-220
- Carruthers, D. J., 2003, Modeling of secondary petroleum migration using invasion percolation techniques, in S. Du¨ppenbecker and R. Marzi, eds., Multidimensional basin modeling, AAPG/Datapages Discovery Series No. 7, p. 21–37.
- Furre, A.K., Eiken, O., Alnes, H., Vevatne, J.N. and Kiær, A.F. [2017]. 20 years of monitoring CO2-injection at Sleipner. Energy Procedia, 114, pp. 3916-3926.
- Hefny, M., Qin, C., Saar., and Ebigbo, A., 2020. Synchrotron-based pore-network modeling of two-phase flow in Nubian Sandstone and implications for capillary trapping of carbon dioxide. International Journal of Greenhouse Gas Control 103.
- Reynolds, C.A. and Krevor, S. [2015]. Characterizing flow behavior for gas injection: Relative permeability of CO-brine and N2-water in heterogeneous rocks. Water Resources Research, 51(12), 9464-9489.
- Trevisan, L., Pini, R., Cihan, A., Birkholzer, J.T., Zhou, Q., GonzálezNicolás, A. and Illangasekare, I. [2017]. Imaging and quantification of spreading and trapping of carbon dioxide in saline aquifers using meter-scale laboratory experiments. Water Resources Research, 53, pp. 485-502.